Introduction to Field-Effect Transistors (FETs)
Field-Effect Transistors (FETs) are key in modern electronics. They control current flow in a semiconductor using an electric field. These transistors are widely used. They have high input impedance and low power usage. This makes them essential in many analog and digital circuits.
Brief History and Development of FETs
The Field-Effect Transistor was first proposed in the 1920s. But it wasn’t until the mid-20th century that the tech was developed. FETs became popular. They enabled efficient amplification and switching with little energy loss. They have evolved into types like the MOSFET and the JFET. Each has unique traits for different uses.
What is a Field-Effect Transistor (FET)? Overview and definition
A Field-Effect Transistor is a three-terminal device. It regulates the current flow using an electric field. It has a semiconductor channel. Current flows between two electrodes: the source and drain. A third electrode, the gate, controls the current. FETs are more efficient than traditional transistors. Their gates control current flow without direct contact. This is important in weak-signal amplification.
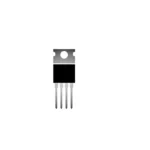
Importance of FETs in Modern Electronics
In modern electronics, FETs play a crucial role due to their versatility in both analog and digital applications. They are widely used in amplifiers, voltage regulators, and switches. This is especially true for portable devices where low power use is vital. The development of MOSFETs has driven the miniaturization of devices and circuits. So, FETs are a cornerstone of modern technology.
Types of Field-Effect Transistor
Field-Effect Transistors (FETs) are essential components in modern electronics. They are widely used due to their ability to efficiently control current flow. There are several types of FETs, each with distinct characteristics and applications.
Junction Field-Effect Transistor (JFET)
The Junction Field-Effect Transistor (JFET) is one of the simplest types of FETs. It controls the current flow through a channel. A voltage applied to the gate terminal does this. The JFET can be n-channel or p-channel. It depends on the type of charge carriers (electrons or holes) that flow through the channel. JFETs are often used in low-noise amplifiers. They have a high input impedance.
Metal-Oxide-Semiconductor Field-Effect Transistor (MOSFET)
The Metal-Oxide-Semiconductor Field-Effect Transistor (MOSFET) is the most used FET in modern electronics. MOSFETs are popular in ICs and power devices. They have low power use and high switching speed. Enhancement mode and depletion mode are their two varieties. They are available in both n-channel and p-channel varieties. The MOSFET is highly versatile and is used in both digital and analog circuits.
Other Types: MESFET, HEMT, and more
Other FETs include the MESFET and HEMT. The MESFET (Metal-Semiconductor Field-Effect Transistor) is for microwave and high-frequency use. The HEMT (High Electron Mobility Transistor) is common in high-speed devices. These advanced FETs meet strict performance needs in fields like telecom and radar.
Working of Field-Effect Transistor (FET)
FETs use an electric field to control current in a semiconductor. They are ideal for applications that need low power, efficient switching, and amplification.
Working Principle: The Role of Electric Fields in Controlling Current Flow
In an FET, the flow of current between the drain and source terminals is regulated by an electric field. A voltage at the gate terminal creates this field. It enhances or restricts the flow of charge carriers (electrons or holes) in the channel. FETs use a single carrier type, unlike bipolar transistors. They rely on both electrons and holes. FETs have simpler operation and lower noise.
Key Components and Structure
The three main components of an FET are the gate, drain, and source. The source is where the current enters. The drain is where it exits. The electric field in the channel is adjusted by the gate. Varying the gate voltage controls the channel’s conductivity. This modulates the current flow between the source and drain.
High Input Impedance and Low Power Consumption Advantages
FETs are renowned for drawing relatively little current from the driving source due to their high input impedance.
This makes them extremely efficient in terms of power consumption. Also, they have low power dissipation. This makes them suitable for battery-powered and low-noise devices. The high input impedance also contributes to the reduced signal distortion in amplifiers.
Construction of Field-Effect Transistor
A Field Effect Transistor (FET) is a type of semiconductor device that controls current flow through a channel via an electric field. Its construction involves several key components:
Channel: The core of the FET, made of semiconductor material (either n-type or p-type), forms the pathway for current. The channel connects the source and drain terminals.
Terminals:
The terminal where the current enters the FET. It supplies carriers (electrons for n-type or holes for p-type).
Drain: The terminal where the current exits the FET.
Gate: This terminal controls the channel’s conductivity by applying a voltage. This creates an electric field that modulates the channel’s width and current flow.
Insulation: In many FET types, the gate is insulated from the channel. It often uses silicon dioxide. This prevents current from flowing to the gate but allows control over the channel.
Substrate: The base material of the FET, usually a silicon wafer, provides support.
The design may vary based on the type of FET, like a Junction FET (JFET) or a Metal-Oxide-Semiconductor FET (MOSFET). They have unique construction details but follow this general framework.
Applications of FETs
Field-Effect Transistors (FETs) are versatile devices. They are used in digital and analog circuits. They are efficient, quiet, and consume little power. Below are key applications of FETs:
Amplifiers, switches, and voltage regulators
FETs are widely used in amplifiers. They have a high input impedance, which minimizes the load on the input source. They are used in low-noise audio, RF, and instrumentation amplifiers. Also, FETs are often used as switches in digital circuits. They control current flow with an applied voltage to the gate. FETs are also vital in voltage regulators. They ensure a stable output in power supplies.
Use in Digital and Analog Circuits
FETs are crucial components in both digital logic circuits and analog signal processing. They can act as electronic switches. So, they are key to modern digital systems, like microprocessors and memory chips. FETs are used in analog circuits for amplification and filtering. They require high-speed switching and low distortion.
Role in Low-Power and High-Speed Devices
FETs are ideal for low-power, portable devices like smartphones and laptops. Their fast switching makes them suitable for high-speed devices, like RF systems and fast digital circuits.
Advantages of FETs
Field Effect Transistors (FETs) have several advantages. They make FETs preferable for many electronic applications.
High Input Impedance: FETs have an exceptionally high input resistance, often in the megaohm range. This feature reduces the loading effect on prior circuits. It helps them work better.
Low Noise: FETs are less noisy than BJTs. This makes them ideal for high-signal apps (like audio and RF amplifiers).
Lower Power Consumption: FETs usually use less power than BJTs. This is critical for battery-operated devices and energy-efficient designs.
Easier Fabrication: FETs are easier to fabricate. They allow for higher chip packing densities. This contributes to the miniaturization of electronic components.
Simple Biasing Requirements: FETs are easier to bias than BJTs. This simplifies circuit design and improves reliability.
Advancements in FET technology
Recent FET innovations, especially with 2D materials, have impacted modern electronics. Materials like graphene and molybdenum disulfide (MoS₂) have boosted FET performance. They have high carrier mobility and better scalability. The use of 2D materials in FETs is revolutionizing devices by making them more efficient, compact, and faster.
Recent Innovations: 2D Materials for FETs
A key innovation is 2D material-based FETs. They are more sensitive and flexible. These FETs are now used in sensors and wearable biosensors for real-time healthcare monitoring. Their ability to detect tiny changes makes them ideal for healthcare and chemical detection systems.
Impact on Modern Electronics
2D material-based FETs impact smartphones and other high-speed devices. They need low power usage and fast switching. FETs in these devices enable high-speed data processing. They improve the user experience in smartphones and sensors that monitor the environment and industry.
Future Trends in Field-Effect Transistor (FET)
As Field-Effect Transistors (FETs) evolve, new technologies are reshaping their development. A thrilling advancement is the use of 2D materials like graphene in FETs. It improves their speed, efficiency, and scalability. FETs are vital in cutting-edge industries. They need high-performance transistors for miniaturization and power optimization.
Emerging Technologies and Their Potential Impact
FETs are now being used in combination with quantum computing and AI technologies. The rising demand for fast data processing and low power use favors 2D material-based FETs. These FETs could improve qubit manipulation in quantum computers. They might help with error correction and coherence time issues.
Future Applications in Quantum Computing and AI
The future of FET technology is particularly promising in the realm of AI and quantum computing. Integrating FETs into AI systems can enable faster, more efficient computing. Also, in quantum computing, FETs can optimize qubit performance. This will make the tech more practical for real-world uses, like cybersecurity and advanced machine learning.
Conclusion
Summary of the Importance and Evolving Role of FETs in Electronics
FETs are now vital in modern electronics. They can precisely control current flow while using little power. FETs are better than traditional bipolar junction transistors. They switch faster, are more efficient, and integrate better into semiconductor tech. They are essential in modern electronics. They are used in many applications, from signal amplification to power regulation. FETs are now versatile. They support new fields like biosensing and flexible electronics.
Future Outlook and Ongoing Research
FET technology has a bright future. Research is focused on new materials. These include 2D materials like graphene and organic semiconductors. They could revolutionize FETs in flexible and wearable electronics. Also, using FETs in advanced tech like quantum computing and AI could boost speed and energy efficiency. Research is also exploring biosensors. FETs could detect biological molecules with high sensitivity. This may open new possibilities in medical diagnostics.
Read more: Cooling Method of a Transformer