Introduction to Power Factor
Efficient electrical systems rely on power factor. This key metric measures energy use efficiency. A high power factor indicates good efficiency. In contrast, a low power factor means waste. Understanding power factor is crucial for anyone in the electrical field. It affects costs and performance. Knowing power factor improvement methods reduces energy loss and boosts efficiency. Smart power management begins here.
Energy efficiency is vital today, not just a trend. Improving power factor is key. Engineers, energy managers, and cost-savvy people should learn these power factor improvement methods. This guide covers effective techniques of power factor improvement methods. It helps readers make informed decisions and use energy better. Mastering these power factor improvement methods leads to savings and better system performance.
Understanding Low Power Factor
Low power factor is more common than you might think. But what causes it? The main culprits are inductive loads like motors, transformers, and some lights. These devices need a magnetic field to work. So, they use reactive power. Idle reactive power adds to the electrical load. But, it does no useful work. It’s a phantom force, silently shaping the grid’s total power demand.
Effects of Low Power Factor
Low power factor hikes up electricity costs. Its ripple effects spread wide. They impact more than just your wallet. Utilities often charge extra fees for a low power factor. It stresses the electrical grid. Also, a low power factor increases the system current. This causes more losses and reduces the capacity for extra loads. This inefficiency can cause overheating and wear in electrical parts. Equipment deteriorates faster, hiking upkeep expenses and curtailing longevity. This double whammy strains budgets and operations alike.
Advantages of Power Factor Improvement
Why should you care about power factor improvement? The benefits are numerous and impactful.
Energy Efficiency: Improving the power factor boosts efficiency. It cuts waste and maximizes output. This optimization not only conserves power but also bolsters your electrical system’s reliability. By improving performance, you’ll free up energy for work. This will create a leaner, more effective setup.
Cost Savings: Another significant benefit is cost savings. Many utilities penalize low power factors. Improving it can help you avoid these charges. Also, a higher power factor may lower your electricity bill. Big companies earn huge profits. Their energy-saving actions build over time. Small changes lead to big benefits. Efficiency grows, cutting costs each year. Wise decisions now lead to future rewards.
Reduced Carbon Footprint: Energy efficiency slashes consumption and emissions. Improving your power factor reduces your carbon footprint. It also helps global sustainability. This efficient use of power is vital for a greener Earth. It helps fight climate change, one household at a time.
Power Factor Improvement Methods
Improving power factor offers key benefits. For instance, consider capacitor banks, synchronous motors, or electronic correction devices. Also, regularly maintain equipment and upgrade inefficient motors. These strategies can greatly boost system efficiency and cut costs.
Capacitor Banks
Power factor enhancement often relies on capacitor banks, a widely adopted solution.
What Are Capacitor Banks?
Capacitor banks are groups of connected capacitors. They store and release electrical energy. In an electrical system, they provide leading reactive power. This counteracts the lagging reactive power from inductive loads.
How Capacitor Banks Improve Power Factor
When installed, capacitor banks inject leading reactive power into the system. This offsets the lagging reactive power, effectively raising the power factor. The result? Your system becomes more efficient, reducing energy losses and lowering electricity costs.
Types of Capacitor Banks
There are generally two types of capacitor banks: fixed and automatic. Fixed capacitor banks are manually switched on or off. They work best for systems with stable loads. Automatic capacitor banks adjust their output based on the system’s power factor. They are ideal for systems with varying loads. They provide flexibility and optimal power factor correction.
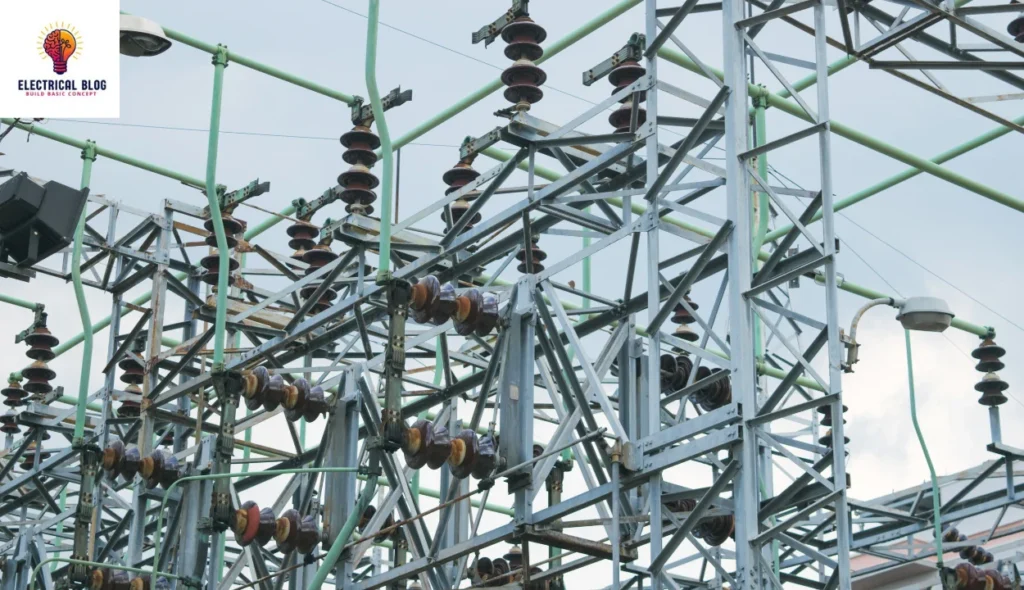
Advantages of Capacitor Banks
Low losses: Capacitors have low internal losses, making them energy-efficient.
Minimal Maintenance: With no moving parts, capacitor banks require very little maintenance.
Easy Installation: Capacitor banks are lightweight. They do not need a complex foundation for installation.
Disadvantages of Capacitor Banks
Limited Lifespan: Most capacitors last 8 to 10 years before needing replacement.
Switching surges: Frequent capacitor bank switching risks surges. Limit on-off cycles to minimize this hazard.
Capacitors are sensitive to voltage changes. If the voltage exceeds rated limits, it can damage them.
Synchronous Condensers
Synchronous condensers are great for correcting the power factor. They work well in large industrial settings.
Overview of Synchronous Condensers: Spinning like a motor, the synchronous condenser hums. Its purpose is to stabilize power grids with reactive power. This rotating machine, though motionless, plays a vital role in electrical systems. It runs without a mechanical load. Its sole purpose is to improve the power factor by providing or absorbing reactive power.
How They Work in Power Factor Correction
Synchronous condensers can change their reactive power output. They do this by varying the motor’s excitation. When the system needs more reactive power, the condenser provides it. Conversely, it can absorb excess reactive power if the system has too much. This dynamic adjustment makes synchronous condensers highly effective for real-time power factor correction.
Advantages of Synchronous Condensers
Longevity: For decades, synchronous condensers have reliably served power grids. These robust machines typically last over 25 years. They have very reliable electrical systems.
Stepless Adjustment: DC excitation adjustments allow fine-tuning of the power factor. By modifying excitation levels, engineers can optimize system efficiency and performance.
No Harmonics: Synchronous condensers are free of harmonic distortion. They differ from some electronic devices.
Disadvantages of Synchronous Condensers
High Initial Cost: Installing and maintaining a synchronous condenser is expensive. So, it suits only large power users.
Auxiliary Equipment Required: Synchronous condensers need an auxiliary starting mechanism. They have no self-starting torque.
Phase Advancers
For systems with induction motors, phase advancers improve power factor. They’re a practical method to do so.
Introduction to Phase Advancers
Phase advancers are devices. They improve the power factor of induction motors. They do this by shifting the current phase closer to the voltage.
This reduces the amount of lagging reactive power drawn by the motor. Their role in power factor improvement. Installing phase advancers, which will greatly reduce your system’s reactive power. This results in a higher power factor. It makes your induction motors more efficient. It’s a simple, cheap fix for systems that rely on these motors.
Advantages of Phase Advancers
Reduce Lagging Reactive Power: Pairing motors with capacitors cuts their lagging reactive power. This boosts system efficiency.
Applicability in Specific Situations: They are very useful when synchronous motors are impractical.
Disadvantages of Phase Advancers
Phase advancers prove costly for small motors. Their benefits only justify use in larger systems exceeding 200 HP or 150 kW. Below this threshold, the investment rarely pays off.
Static VAR Compensators (SVC)
Static VAR Compensators (SVC) are advanced devices. They manage reactive power in high-voltage power systems.
What is SVC?
An SVC is a system that uses power electronics to control reactive power dynamically. It can inject or absorb reactive power as needed. This makes it very versatile and responsive to changing system conditions.
How SVC Improves Power Factor: SVCs precisely control the power factor. This is vital in systems with fluctuating loads. They improve the electrical system’s efficiency and stability. They do this by adjusting the reactive power to maintain an optimal power factor.
Advantages of SVCs
Dynamic Response: SVCs can quickly adjust reactive power to match changing loads.
Harmonic Filtering: SVCs can also function as harmonic filters, improving overall power quality.
Disadvantages of SVCs
SVCs are complex and costly to install and maintain. They need advanced control systems.
Automatic Power Factor Correction (APFC) Panels
APFC panels are common in industrial and commercial settings. They automatically correct the power factor.
What Are APFC Panels?
APFC panels are advanced devices. They monitor an electrical system’s power factor in real time. They automatically switch capacitor banks in and out of the circuit. This maintains the desired power factor.
How APFC Panels Optimize Power Factor: They are ideal for systems with varying loads. They continuously measure the power factor and adjust the capacitors accordingly. They keep the power factor optimal, maximizing efficiency and cutting costs.
Choosing the Right Power Factor Improvement Methods
Choosing the right power factor improvement methods takes careful thought. First, assess your needs. Then, review options and their pros and cons. Consider costs, time limits, and goals. Seek advice from experts or peers. Finally, pick the power factor improvement methods that best matches your goals and resources. It comes down to understanding your specific requirements and constraints.
Factors to Consider
Type of Load: The type of load in your system is a crucial factor. For example, if your system uses motors, phase advancers might be the best choice.
System Size: Larger systems might benefit more from SVCs or APFCs. They offer better, more precise control.
Cost: Some methods, like synchronous condensers, can be expensive. But they often save money long-term through better efficiency and less maintenance.
Cost vs. Efficiency
Balancing costs with productivity is key. Efficiency must not break the bank, yet budgets shouldn’t stifle results. The sweet spot lies in smart resource allocation. SVCs and APFC panels offer precise control. But they have high initial costs. However, long-term savings on energy bills can offset these costs. They will also reduce demand charges.
Industrial vs. Residential Applications
The application is key in choosing the right method. Industrial systems have large, variable loads. They often need more advanced solutions, like SVCs or APFC panels. In contrast, residential systems might only need capacitor banks to fix the power factor.
Implementation of Power Factor Correction
Plan carefully for power factor correction. Execute your plan precisely. Success relies on good preparation and execution. Pay attention to detail for the best results. A solid plan ensures smooth implementation.
Steps for Implementing Power Factor Correction
Assessment: First, analyze the current power factor. Then, identify the causes of the low power factor in your system.
Selection: Choose the best power factor correction method for your system. It should fit your needs and constraints.
Installation: Qualified professionals should handle installation. Follow manufacturer guidelines precisely. This ensures proper setup and prevents potential problems with the chosen equipment.
Testing: Test the newly installed system for proper operation. Verify that the power factor improvement meets expectations. Ensure all components function correctly.
Common challenges and how to overcome them
Incorrect Sizing: One of the most common challenges is incorrect sizing of the power factor correction equipment. Make sure to size the equipment accurately based on your system’s needs.
Overcompensation: Overcompensation, where the power factor becomes leading, can also be a problem. Avoid this by choosing the right method and regularly monitoring the system.
Maintenance: Expenses rise and inefficiency is fostered by neglect. On the other hand, a well-maintained system functions efficiently and economically. The secret to peak performance and long-term cost reduction is regular maintenance.
Monitoring and Maintenance of Power Factor Correction Systems
After your power factor correction system runs, it needs regular checks and upkeep. This will ensure its longevity and effectiveness.
Regular monitoring is important. It lets you find issues in the power factor correction system early. This can stop problems from growing and keep your system efficient. Regular monitoring helps find changes in the load. These may require adjustments to the correction equipment.
Maintenance Tips for Longevity
Periodic Inspections: Begin with switches, then move to capacitors and wiring. Examine each part closely for damage. Regular checks ensure system longevity. Watch for wear indicators throughout the inspection process.
Cleaning: Maintain pristine gear, banishing dust and grime. This helps to maintain optimal performance.
Testing: Test the system periodically. It should improve the power factor as expected. Performance dips? Consider upgrading or swapping out parts. A timely refresh can revitalize your system’s speed and efficiency.
Conclusion
Improving your power factor is more than just a technical exercise. It is vital. It will make your electrical system more efficient. It will cut energy costs and help build a sustainable future. By using the right power factor improvement methods, you can optimize your system. It will reduce your electricity bills and extend your equipment’s life.
Power factor improvement methods like Capacitor banks, synchronous condensers, and phase advancers each have unique benefits. So do more advanced solutions like SVCs and APFC panels. The key is to assess your needs, select the right power factor improvement methods, and implement it carefully. With regular upkeep, you’ll enjoy a better power factor for years.